Solar Radiation & Photosynthetically Active Radiation
What is Solar Radiation?
Solar radiation is radiant (electromagnetic) energy from the sun. It provides light and heat for the Earth and energy for photosynthesis. This radiant energy is necessary for the metabolism of the environment and its inhabitants 1. The three relevant bands, or ranges, along the solar radiation spectrum are ultraviolet, visible (PAR), and infrared. Of the light that reaches Earth’s surface, infrared radiation makes up 49.4% of while visible light provides 42.3% 9. Ultraviolet radiation makes up just over 8% of the total solar radiation. Each of these bands has a different impact on the environment.

The amount and intensity of solar radiation that a location or body of water receives depends on a variety of factors. These factors include latitude, season, time of day, cloud cover and altitude. Not all radiation emitted from the sun reaches Earth’s surface. Much of it is absorbed, reflected or scattered in the atmosphere. At the surface, solar energy can be absorbed directly from the sun, called direct radiation, or from light that has been scattered as it enters the atmosphere, called indirect radiation 1.
How is Solar Radiation Measured?
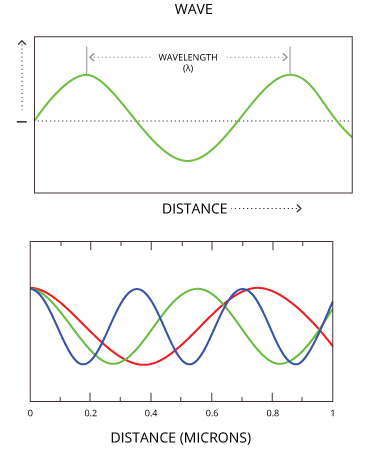
Solar radiation is measured in wavelengths or frequency. As light travels in a wave, a wavelength is defined as the distance from peak to peak and is measured in nanometers (nm). Frequency is defined as wavelength cycles per second and is expressed in hertz (Hz). Bands with shorter wavelengths produce higher frequencies. Likewise, the longer the wavelength, the longer it will take to complete a cycle, which produces a lower frequency 1.
The energy of the wavelength increases with the frequency and decreases with the size of the wavelength 16. In other words, shorter wavelengths are more energetic than longer ones. This means that ultraviolet radiation is more energetic than infrared radiation. Due to this extra energy, shorter wavelengths tend to cause more harm than longer wavelengths 16. The more energy a wavelength has, the easier it is to disrupt the molecule that absorbs it. Ultraviolet light (which has the highest energy) can cause damage to DNA and other important cellular structures 16.
What is the Electromagnetic Spectrum?
The electromagnetic spectrum encompasses all types of radiation 5. The part of the spectrum that reaches Earth from the sun is between 100 nm and 1 mm. This band is broken into three ranges: ultraviolet, visible, and infrared radiation. Ultraviolet contains wavelengths between 100-400 nm. Visible light falls within the range of 400-700 nm, and infrared light contains wavelengths from 700 nm to over 1 mm 1. In the visible light spectrum, the colors are determined by the length. Longer wavelengths appear red while shorter wavelengths are blue/violet as they range closer to the ultraviolet spectrum 5.
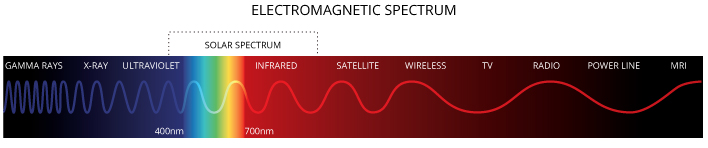
Ultraviolet Radiation
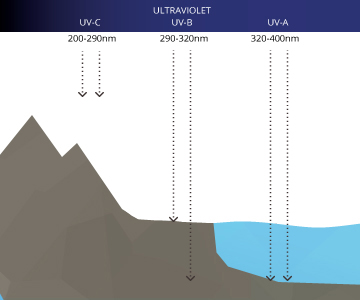
Ultraviolet radiation can be separated into three wavelength ranges: UV-A, UV-B and UV-C. All wavelengths of ultraviolet light can directly affect the DNA of water inhabitants as well as generate harmful photochemicals 1. The shorter the wavelength, the more damage it is capable of causing.
UV-C includes wavelengths between 100 and 280 nm. This radiation range only makes up 0.5% of all solar radiation, but it can cause the most damage to organisms. However, most of this short-wave radiation is absorbed by stratospheric gases (ozone), and very little reaches the surface 9.
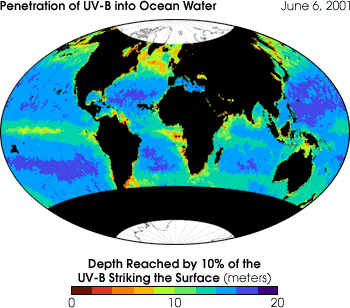
UV-B (280-320 nm) is an energetic, photoactivating band of radiation that is only partially absorbed in the stratosphere 1. This radiation band is known for causing skin cancer in humans, and can impair photosynthesis in many plants 16. The depth that UV-B penetrates water is dependent on turbidity and water chemistry. UV-B will reach greater depths in saline water than in freshwater and can reach as deep as 20m below the surface of the ocean 1,9.
UV-A (320-400 nm) has less energy than UV-B, and is not absorbed by ozone in the atmosphere. However, it can be blocked from the surface by cloud cover 9. UV-A is also called blacklight, and is known for its ability to cause fluorescence in some materials 37. While it is absorbed less readily by water, it can penetrate deeper than UV-B or UV-C 1. UV-A is responsible for sunburns in humans. It is also more inhibiting than UV-B in regards to photosynthesis 1. Studies have shown that UV-A can significantly decrease photosynthesis by more than 70% 6. This is because UV-A decreases the efficiency of electron transport which in turn decreases photosynthetic production.
Ultraviolet Radiation and Phytoplankton
Phytoplankton are microscopic organisms that reside in water and use photosynthesis to convert sunlight to energy 16. These organisms use carbon dioxide and produce oxygen as a photosynthesis byproduct just as plants do 17. Ultraviolet light can stunt this process in phytoplankton. UV-A and UV-B radiation inhibit photosynthetic production, thus reducing carbon dioxide intake and oxygen output. Under natural sun-lit conditions, UV-A and UV-B can diminish photosynthesis by over 8% 41.
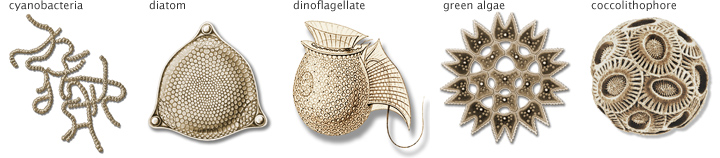
This effect can be detrimental to more than just phytoplankton. These one-celled plants are responsible for much of the carbon transfer that occurs between the atmosphere and the ocean, a process known as the “biological carbon pump” 17. Much of the ocean life below the surface depends on phytoplankton, consuming them directly or indirectly 17. Phytoplankton also contribute to “marine snow” – the dead, organic material that falls to the ocean floor as fuel for deep sea organisms. When ultraviolet radiation reduces photosynthetic production of phytoplankton, it negatively affects the world carbon cycle and the marine food chain 16.
Infrared Light

Infrared light is on the opposite side of the spectrum from ultraviolet light. This radiation has a wavelength of >700 nm and provides 49.4% of solar energy 9. Infrared radiation is readily absorbed by water and carbon dioxide molecules and converted to heat energy 10. The longer wavelengths cause heat by exciting electrons in the substances that absorb them. Thus infrared radiation is responsible for warming Earth’s surface. Infrared light is reflected more than UV or visible light due to its longer wavelengths 10. This reflection allows infrared radiation to transfer heat between the surface, water and the air.
In a body of water, infrared light can only reach a certain distance below the surface. 90% of infrared radiation is absorbed in the first meter of the water’s surface, and only 1% can reach past two meters in pure water 1. This is why the surface of most bodies of water are warmer than the depths.
What is Photosynthetically Active Radiation?
Photosynthetically Active Radiation (PAR) is the light wavelength range that is best fit for photosynthesis to occur. Photosynthesis is a process that requires light energy and optimally occurs in the 400 to 700 nanometer (nm) range 1. This range is also known as visible light.
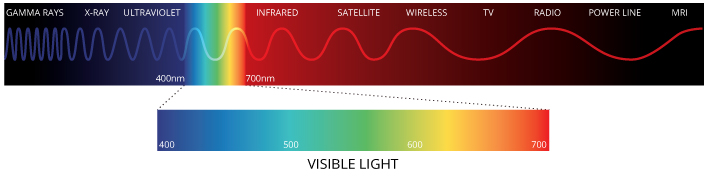
Visible light encompasses the electromagnetic spectrum from visible blue/violet to red. Blue light has a higher energy and shorter wavelength than green or red light. Red light has the lowest energy in the visible spectrum 12. As visible light reaches Earth, a surface will absorb or reflect different wavelengths, producing a visible color. The wavelength reflected by a surface is the color that it appears to be 12. If the surface reflects all of the visible wavelengths, it will appear white 12.
Most plants appear green as the chlorophyll in their cells reflects green light 8. Water often appears blue as this color travels the deepest before being absorbed 1. While on land, plants use nearly all of the visible range for photosynthesis. However, even underwater when only blue light is available, photosynthesis can still occur.
Why are Solar and Photosynthetically Active Radiation Important?
Solar radiation provides heat, light, and energy necessary for all living organisms. Infrared radiation supplies heat to all habitats, on land and in the water 24. Without solar radiation, Earth’s surface would be about 32°C colder 25.
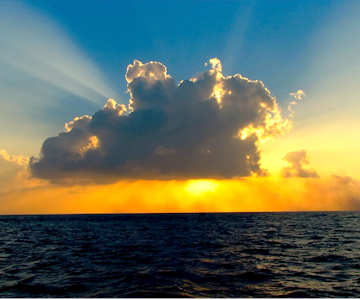
Light is also provided by solar radiation. Predators would not be able to efficiently hunt prey without light from the sun and prey would not be able to take advantage of dark areas if predators were adapted to dark habitats 1. Human eyes are adapted to the visible spectrum, though some other species can see ultraviolet light in addition to colors 26.
In particular, the level of photosynthetically active radiation (PAR) that an area receives is important. This is because different plants respond to different wavelengths of PAR 1. Most plants reflect green wavelengths while absorbing the rest of the visible light spectrum. In addition, shade plants respond to lower levels of PAR while sun plants harvest PAR more efficiently at higher light levels 7. In other words, as solar irradiance (intensity) increases, sun plants experience higher rates of photosynthesis. The leaves of sun plants are small and thick, with special cells allowing for these higher rates 20. Shade plants conduct photosynthesis at a lower radiation intensity level. Their leaves are thinner, longer and contain fewer chlorophyll cells. This makes it easier for photosynthesis to occur in low light conditions 20.
Although the main benefit of photosynthesis is energy for the plant, it has other important results. Oxygen is a byproduct of photosynthesis 1. The process ensures that more oxygen is produced than is used up by organisms in the surrounding environment. If photosynthesis does not produce enough dissolved oxygen underwater, it can create anoxic conditions where fish and other organisms cannot live 1. Photosynthesis also consumes carbon dioxide, thus lowering carbon dioxide levels in air and water 1.
Solar Irradiance
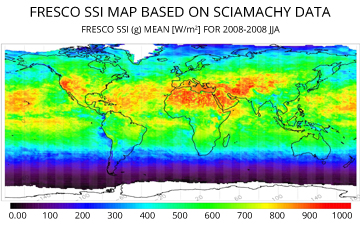
Solar irradiance is the intensity with which radiation enters Earth’s atmosphere. An relatable way to think about solar irradiance is by looking at the difference between a 20-watt light bulb and a 100-watt light bulb. Both produce visible light in the same wavelengths, but the brightness and intensity are very different. The 100-watt bulb has a higher intensity, or irradiance. Solar irradiance is the amount of radiant flux on an area, and is measured in watts per meter squared (W/m²) 9.
What Influences Solar Irradiance?
The solar irradiance received by a particular location or body of water depends on the elevation above sea level, the angle of the sun (due to latitude, season and time of day) and scattering elements such as clouds 9. The higher the elevation, the shorter the path from the atmosphere. This can mean a higher irradiance, though not warmer temperatures. This intense radiation contributes to the arid climates, and the thinner air means more UV radiation reaches the surface at these altitudes.
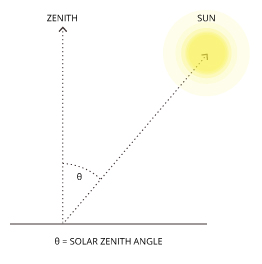
The lower the angle of the sun, the larger amount of ozone the light has to pass through 9. This is also factor in ultraviolet irradiance. Ozone absorbs UV light and can reduce radiation intensity.
The angle of the sun is dependent on latitude, time of year, and time of day. The distance that radiation has to travel will be at its lowest when the sun is directly overhead. This is why the annual net solar irradiance is greater over the equator than over the northern and southern latitudes. Solar irradiance will decrease as a hemisphere is tilted away from the sun.
During the day, the sun’s angle to any given location will decrease from sunrise until noon, and then increase until sunset. At greater angles (morning and evening) solar radiation has to pass through more of the atmosphere, which reduces its irradiance. This is why sunlight feels less intense in the evening than at noon.
Cloud coverage and air pollution can also reduce the amount of radiation that reaches Earth’s surface. Clouds and aerosols in the atmosphere can scatter and absorb all radiation bands 9. As cloud cover increases, the angle of the sun becomes less important when measuring irradiance. This is due to the increase of radiation diffusion (scattering) 10. Increased cloud cover decreases irradiance, causing sunlight to feel less intense. On these days solar radiation still reaches Earth’s surface, simply with a lower irradiance. Under these conditions, humans can become sunburned without realizing the effects until it is too late.
Heat, Temperature and Solar Radiation
Sunlight is responsible for warming the Earth, oceans and atmosphere through infrared radiation. Both water and land reflect back some of that radiation to warm the atmosphere or other objects in contact with the surface. The darker the object or surface, the faster it will absorb light and heat 31.
Air Temperature
Air temperature is indirectly dependent on solar radiation. While air itself does not absorb infrared radiation, it receives heat from Earth’s surface. This effect occurs through heat transfer by conduction and convection 31.
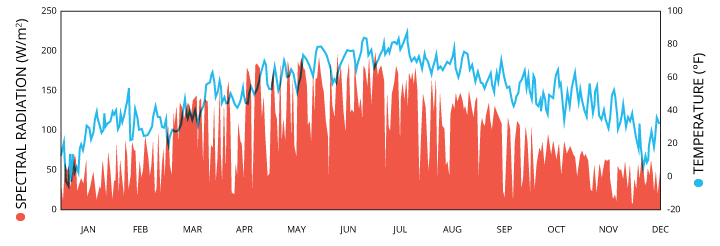
Earth absorbs infrared radiation and converts it to thermal energy. As the surface absorbs heat from the sun, it becomes warmer than the surrounding atmosphere. The heat is then transferred by conduction (contact) from the warmer Earth to the cooler atmosphere 24. Air itself is a poor conductor of heat, so convection, or the rise and fall of warm and cool air, warms the rest of the atmosphere not in contact with the surface 31. The rising warm air is often referred to as a thermal. As the warmed air rises, cooler air sinks to the surface, where it continues in the convection process.
Earth’s surface also reflects some infrared radiation back into the air. This reflected radiation can be trapped and absorbed by gases in the atmosphere, or re-radiated back to the Earth 25. This process is called the greenhouse effect. Without the greenhouse effect, the Earth’s average surface temperature would be about-18°C instead of the current +18°C 25.
Water Temperature

Infrared light from the sun is absorbed by bodies of water and converted to heat energy. This low energy radiation excites electrons and warms the top layer of water. Nearly all infrared radiation is absorbed within one meter of the surface 1. This heat is then transferred to greater depths through movement from wind and convection 1. While heat is slowly transferred throughout the water column, it often does not reach all the way to the bottom. This is due to water column stratification.
In the ocean and many lakes, water can stratify, or form distinct layers of water. These layers are distinguished by their temperatures, densities and often different concentrations of dissolved substances (such as salt or oxygen). The different water strata are separated by steep temperature gradients known as thermoclines 1. Even with convection and wind, it is difficult for most of the sun’s heat to cross these barriers. Instead, the lowest strata of water will remain near 4°C, while the surface water temperature will fluctuate both diurnally (daily) and seasonally 1.
What is Photosynthesis?
Photosynthesis is the process by which plants and other organisms, also known as photoautotrophs, use energy from sunlight to produce glucose. This process can occur both on land and underwater 18.
Glucose is a kind of sugar that is later converted into Adenosine Triphosphate (ATP) via cellular respiration 3. ATP is an energy-bearing molecule that is used in the metabolic reactions of living organisms. This molecule is a necessity in almost all organisms 4. Photoautotrophs use sunlight, six carbon dioxide molecules, and twelve water molecules to produce one molecule of glucose, six oxygen molecules, and six water molecules. This reaction reduces carbon dioxide levels in the air or water while producing glucose for ATP.
Photosynthesis can occur underwater as long as enough light is available. In the ocean, significant amounts of photosynthetically active radiation can be detected as deep as 200 m below the surface 29. Within this euphotic zone (sunlight zone), photosynthesis can occur. This process only requires light, carbon dioxide, and water 18. As long as a photosynthesizing organism, on land or underwater, has enough of these molecules, it can produce glucose and oxygen.
Photosynthesis and Temperature
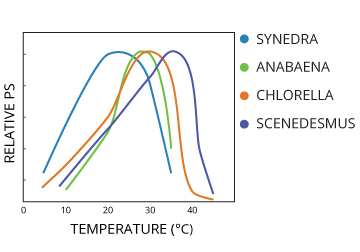
Photosynthesis is a series of chemical reactions that occur with the help of enzymes. Enzymes are catalysts in biological processes and help speed up chemical reactions 11. Photosynthesis also requires heat to activate the process. As heat increases kinetic energy (causing reactants to bump into one another more often), a higher temperature can speed up chemical reactions in addition to initiating the process 11.
Although increased temperatures can speed up photosynthesis, too much heat can be detrimental 11. At a certain temperature, enzymes become denatured and lose their shapes. Denatured enzymes no longer speed up chemical reactions and instead slow down photosynthesis. Thus temperature is an important factor in photosynthetic production, both in activating and maintaining the process. This is why there are different optimal temperatures for photosynthesis for different organisms 1.
How is Underwater Photosynthesis Affected by Turbidity?
Turbidity is a lack of water clarity caused by the presence of suspended particles 1. These particles absorb sunlight and can cause light to be reflected off the particles in water. The more particles present in the water, the less photosynthetically active radiation that will be received by plants and phytoplankton. This loss of sunlight decreases the rate of photosynthesis. If the photosynthetic production is limited, the dissolved oxygen level in the water will decrease 13. In addition, turbidity can cause significant damage to water habitats by absorbing infrared radiation and increasing water temperature above normal levels.
Why can’t Photosynthesis use UV or Infrared Light?
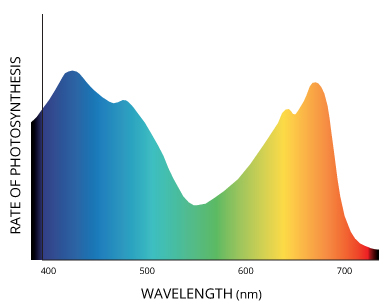
Visible light is the only band of light on the spectrum to be considered photosynthetically active. It has the perfect amount of energy to excite the electrons needed to start photosynthesis and not damage DNA or break bonds.
Ultraviolet can not be used for photosynthesis because it has too much energy. This energy breaks the bonds in molecules and can destroy DNA and other important structures in organisms 8. When plants and other photoautotrophs attempt to use UV-A (320-400 nm) for photosynthesis, electron transport efficiency is decreased, which in turn decreases the rate of photosynthesis 6. On the other side of the spectrum, infrared light does not contain much energy. The insufficient energy does not excite electrons in molecules enough to be used for photosynthesis. Infrared light is converted to thermal energy instead 8.
Typical Solar Radiation Levels
Solar radiation levels are dependent on the time of day and on the sun’s angle toward Earth. This angle will vary by latitude and season. The greater the angle of the sun, the more ozone that sunlight must pass through to reach the surface 9. In addition to the sun’s angle, atmospheric conditions can affect radiation levels. Cloud cover, air pollution and the hole in the ozone layer all alter the amount of solar radiation that can reach the surface. These factors all cause typical radiation levels to differ.
Daily Fluctuations
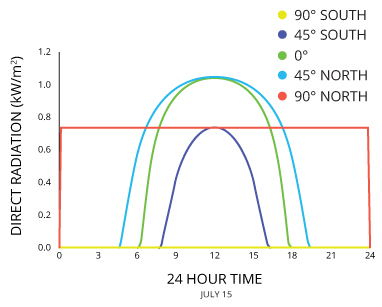
Over most of Earth’s surface, the solar radiation received is measured by the solar irradiance. The irradiance will increase from sunrise until noon, and then decrease until sunset 36. Peak solar energy levels received will vary by latitude and season 15.
As seen on the graph to the left, the equator has the steepest solar radiation curve, giving it the shortest sunrise and sunset periods. In addition, the length of day does not vary greatly throughout the year. This occurs because the angle of the sun does not significantly fluctuate over the equator.
A hemisphere tilted toward the sun would reach a similar peak radiation level as the equator, but with more gradual curves, meaning longer sunrises and sunsets. This hemisphere would also have longer days overall. The opposing hemisphere (tilted away from the sun) would have shorter sunrises and sunsets, as well as shorter periods of daylight 15.
At the geographic North and South Poles (90° latitude), solar irradiance seems to stay constant throughout a single day 15. This is because the poles are the Earth’s rotating point. Although the daily values do not appear to change, the level of solar radiation received at the poles will slowly shift throughout the year.
Monthly Fluctuations
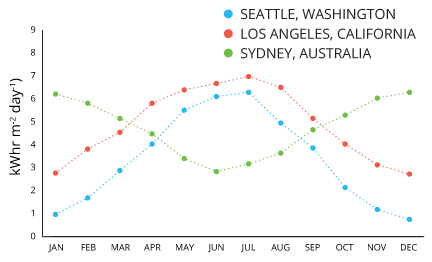
Solar radiation levels depend on proximity to the sun and the sun’s angle. Thus different areas of the globe have different typical radiation levels in each season. At the equator, the typical solar radiation is fairly constant year round 15. There are slight fluctuations but no drastic spikes or drops. In the Northern Hemisphere, the radiation increases as the year progresses until it peaks around June or July. The radiation levels then slowly decrease throughout the rest of the year 14. In the Southern Hemisphere, the radiation levels are opposite. At the beginning of the year, levels are high and then slowly drop to their lowest point around June. After June, they begin to rise again for the rest of the year 14.
The Hole in the Ozone Layer
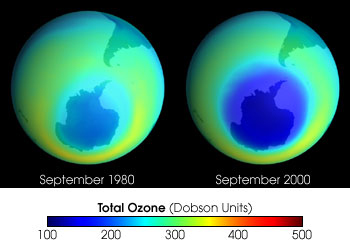
Ozone is a molecular gas composed of three oxygen atoms (O3). This gas helps protect Earth because it absorbs most of the sun’s ultraviolet radiation. The majority of UV-C, most of UV-B and about half of UV-A are absorbed by oxygen and ozone in the ozone layer. This layer is primarily found in the stratosphere, between 10 and 50 km above Earth’s surface.
The “hole in the ozone layer” is found in the atmosphere over the Antarctic. This area is not completely void of ozone, but is instead a patch of atmosphere that possesses a significantly lower level of ozone than normal 27. While the cause of gap is sometimes a subject of debate, studies have shown that ozone is destroyed when it reacts with chlorine, nitrogen, hydrogen, or bromine 27. When these chemicals enter the atmosphere, they can remove the ozone present. Regardless of its cause, the hole in the ozone layer allows more UV radiation to reach Earth. If the increase in UV radiation becomes excessive, it can be harmful to both terrestrial and aqueous habitats 27.
Consequences of Unusual Levels
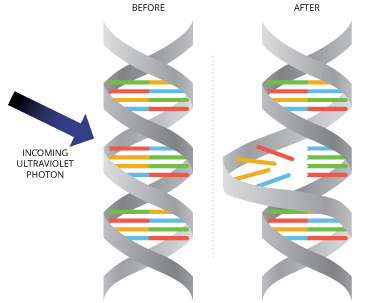
Unusually high or low levels of sunlight can cause problems for both land and water habitats. Too much ultraviolet light can cause irreversible damage to DNA and important photosynthetic structures, while too much infrared light can cause overheating 1. DNA damage is caused by UV-B radiation. While most living cells have adapted and can repair simple damage, increased exposure to UV radiation can cause cells to mutate beyond repair, or to die 16.
On cloudy days, or if a previously sunny location becomes shaded, photosynthetic production can be halted. Not only does this stop oxygen production, but it increases oxygen consumption through plant respiration 1. The decrease in infrared light will also cool the shaded surface or body of water, which in turns cools the surrounding air.
Water
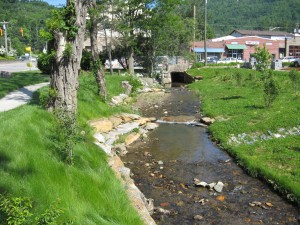
When water is exposed to excessive amounts of sunlight, the infrared radiation will heat the water. The warmer a body water is, the faster the rate of evaporation will be. This can reduce water levels and water flow. In addition, warm water can not hold as much dissolved oxygen as cold water. This means that in warmer water, less dissolved oxygen is available for aquatic organisms 21. Too much infrared light can also cause the enzymes used in photosynthesis to denature, which can slow or halt the photosynthetic process 11.
On the other side of the spectrum, radiation can be limited by cloudy days, shade sources or low sun angles. If radiation from the sun is lower than usual for an extended period of time, photosynthetic production can decrease or be stopped completely. Without sunlight, phytoplankton and plants will consume oxygen instead of producing it. These conditions can cause dissolved oxygen levels in the water to plummet, potentially causing a fish kill 20.
Land
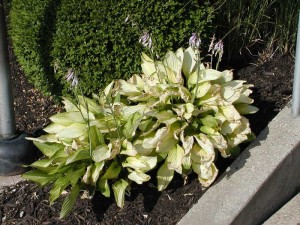
As in water, terrestrial radiation levels can be limited by cloudy weather 20. This is particularly important to plants, as the photosynthetic process and plant physiology in general are dependent on sunlight.
Stomata are pores found on the outer layer of plant leaves. They open in the presence of sunlight and allow water, carbon dioxide, and oxygen to enter the plant 22. These molecules are then used to produce glucose through photosynthesis. On cold, sunless days, stomata close because not enough energy from the sun is being received to continue photosynthesis 23. Too much intense sunlight can also halt the production of photosynthesis, as stomata will close on sunny, hot and dry days to prevent water loss 23.
Sunlight can affect more than the opening and closing of plant stomata. While some plants have specialized proteins that protect them from sunburn, others do not, and intense solar radiation can damage their leaves 32. Plants that are not adapted to full or intense sunlight, such as hostas or rhododendrons, can develop heat stress. Many plants, including shade plants, are susceptible to leaf scorch, where parts of the plant die due to excessive water loss through transpiration 33. In addition to slowing or halting photosynthesis, heat stress and leaf scorch can make plants more susceptible to disease or insect infestations.
How Much Light?

How much light does the sun produce? This is a complex question to answer as there are different ways to consider and measure light. There is radiance (projected power) and visible light, which can be measured as luminance (brightness) or illuminance (incident light). Luminance and illuminance apply only to the wavelengths in the visible light band37. Sunlight is usually defined in radiance units, as only half of the solar radiation that reaches Earth is visible light, but all of the radiation provides energy.
Radiant energy can be measured in joules, though it is more commonly measured as radiant flux, or radiant power, which is expressed as energy over time. The basic unit of power is the watt (joules/second). The sun emits 384,600,000,000,000,000,000,000,000 watts (3.846 x 1026 W) 38. For comparison, the average incandescent light bulb consumes 40-100 watts. This energy is projected out from the sun in a sphere, where some will fall on Earth. The energy that reaches Earth is measured as solar irradiance (energy per second over a square meter). Given the estimated radiant power of the sun, the intensity of solar energy that reaches the top of Earth’s atmosphere (directly facing the sun) is 1,360 W/m² 39.
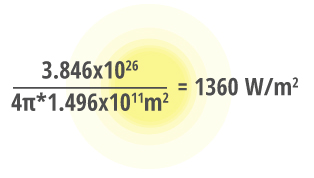
The amount of irradiance that reaches the surface can vary due to Earth’s elliptical orbit, solar flares and the amount of atmosphere the radiation must pass through (due to the sun’s angle to the surface or cloud cover present).
What does that mean in terms of visible light? Luminance and illuminance attempt to define the brightness and the light projected from a given source. A lumen is the standard measure of visible “brightness” as seen by the human eye 37. Lumens can only be measured over the photosynthetically active radiation band, or the range of visible light. One lumen of light over one square meter is one lux (lm/m^2), which is the the SI unit for illuminance. In direct sunlight, when the sun is at its zenith (directly overhead), measured lux could be as high as 130,000 40. On most sunny days (out of direct light), illuminance is usually 10,000-25,000 lux. On an overcast day, incident light may only reach 1000 lux, and at twilight it may be 10 lux 40. The greater the angle of the sun, the lower the lux will be, as the lumens are spread out over a greater area. Illuminance is important to consider when evaluating photosynthetically active radiation.
How much light actually reaches the Earth’s surface?
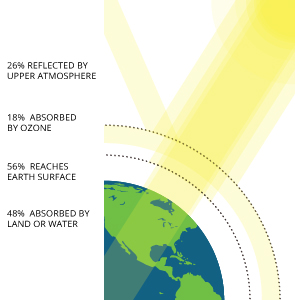
The sun’s radiation must make it through multiple barriers before it reaches Earth’s surface. The first barrier is the atmosphere. About 26% of the sun’s energy is reflected or scattered back into space by clouds and particulates in the atmosphere 34. Another 18% of solar energy is absorbed in the atmosphere. Ozone absorbs ultraviolet radiation, while carbon dioxide and water vapor can absorb infrared radiation 34. The remaining 56% of solar radiation is able to reach the surface. However, some of this light is reflected off of snow or other bright ground surfaces, so only 48% is available to be absorbed by land or water 36. Of the radiation that reaches the surface, approximately half is visible light and half is infrared light 1. These reflection and absorption percentages can vary due to cloud cover and sun angle. In cloudy weather, up to 70% of solar radiation can be absorbed or scattered by the atmosphere 35.
How much light breaks the surface of the water?
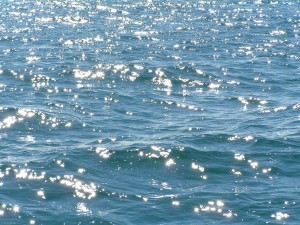
Once the light reaches the surface of the water, reflection and scattering can occur. Reflection occurs when solar radiation simply bounces off the water 1. This reflection is due to water’s albedo, or reflectivity. The amount of sunlight reflected depends on the sun’s angle, wavelength, and weather conditions. Of the light that reaches the water’s surface, approximately 5-10% is reflected 1. Longer wavelengths are reflected slightly more than shorter wavelengths 10.
Scattering is the deflection of light by molecules in the water. Different materials, including dissolved and suspended solids, as well as organisms in the water, cause light to scatter in different directions 1. The amount of light that scatters is dependent on the clarity of the water.
How deep does sunlight reach in the ocean?

The ocean is split into three zones based on light. The first zone, the euphotic or sunlight zone, is where sunlight penetrates. Phytoplankton live in the euphotic zone because there is enough light for photosynthesis. This zone extends to about 660 ft below the ocean surface. 2
The next zone is called the dysphotic (twilight) zone. Some light is able to reach this depth, but it is not enough for photosynthesis to occur 29.
The last zone starts about 3,300 ft below the ocean’s surface and is called the aphotic (midnight) zone. Sunlight cannot reach this zone, and its only light comes from bioluminescent organisms 2.
How deep does sunlight reach in freshwater?
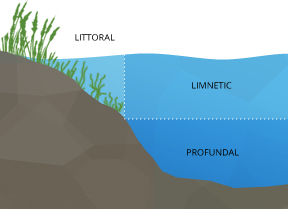
The depth that light penetrates in freshwater is dependent on water clarity. In waters with a high level of turbidity, or suspended solids, light will not reach as far as clear bodies of water. These suspended particles can both absorb and scatter light 1. In most rivers and streams, light will reach the riverbed, and photosynthesis can occur throughout the water column. However, in particularly deep, algae-covered or turbid lakes, light may not be able to reach certain depths.
Like the ocean, deep lakes are split into three zones. The first zone is called the littoral zone. This zone is close to the shore and sunlight reaches all the way to the bottom. Aquatic plants in the littoral zone can grow on the lake bed and still receive enough light for photosynthesis 19. The next zone is known as the limnetic zone and is the surface layer of open water. Photosynthesis can occur in this zone as it is penetrated by light. The depth of the limnetic zone depends on the turbidity of the water. In more turbid water, the limnetic zone will be shallower 19. Below the limnetic zone is the profundal zone. This is the benthic (bottom) layer of a deep lake. Sunlight cannot reach this zone, so photosynthesis will not occur. Instead, organisms that permanently reside in the profundal zone (such as bacteria) rely on falling organic matter from higher zones 19.
Light Transmission Through Ice and Snow
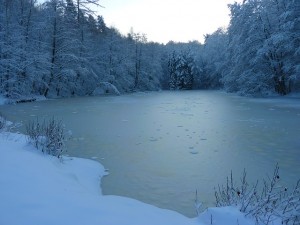
The amount of light absorbed in a body of water can be greatly affected by ice and snow cover. Clear, colorless ice has the same percentage of light transmission as liquid water, which is about 72% 1. However, if the ice is stained or cloudy, the percentage can drop dramatically. Opaque ice and heavy snow can drop the percentage of light transmission to nearly zero. If a body of water is covered in ice and snow for a long period of time, its entire metabolism can be stunted.
Photosynthesis, which requires light, produces oxygen as a byproduct and helps maintain dissolved oxygen levels in a body of water. Dissolved oxygen is continuously consumed in metabolic reactions by living organisms in the water, regardless of snow and ice cover. If snow and ice prevent photosynthesis, plant respiration will contribute to the oxygen depletion instead of restoring dissolved oxygen levels. When this occurs, oxygen reduction, or anoxia, can result and many organisms can die. This often occurs in shallow, productive lakes and ponds and is known as a winterkill 1.
Aquatic Organisms and Visible Light
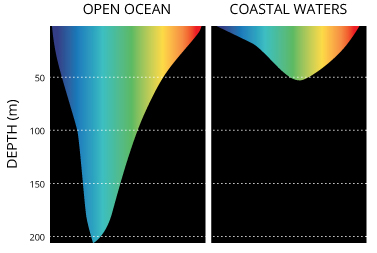
Sunlight can only reach certain depths in water 29. Beyond 200 m, there is not enough light for photosynthesis to occur. The human eye is not sensitive enough to detect sunlight under 850 m of water 26. Even the most visually-adapted fish can not see light from the sun at depths below 1000 m. However, many of the organisms that live at or below this depth still have functioning eyes. Instead of relying on sunlight, they use their own bioluminescence for light 26.
Fish that live near the surface have similar visual abilities to those of organisms on land. They possess color vision because the visible light spectrum penetrates the surface of water26. Many fish are also equipped with UV vision, giving them an opportunity to see animals that are transparent in visible light 26.
Species like shrimp and squid can see the polarization of underwater light in addition possessing color and UV vision. With this ability, they can efficiently locate prey as the polarization of light is altered when it bounces off scales 26. Mantis shrimp may also use this ability for mating, as the male’s paddles change color with a different polarized orientation 30.
Cite This Work
Fondriest Environmental, Inc. “Solar Radiation and Photosynethically Active Radiation.” Fundamentals of Environmental Measurements. 21 Mar. 2014. Web. < https://www.fondriest.com/environmental-measurements/parameters/weather/solar-radiation/ >.
Additional Information
- Measurement Methods
- PAR Sensors
- Underwater PAR Sensors
- Applications
- References