Dissolved Oxygen
What is Dissolved Oxygen?
Dissolved oxygen refers to the level of free, non-compound oxygen present in water or other liquids. It is an important parameter in assessing water quality because of its influence on the organisms living within a body of water. In limnology (the study of lakes), dissolved oxygen is an essential factor second only to water itself ¹. A dissolved oxygen level that is too high or too low can harm aquatic life and affect water quality.
Non-compound oxygen, or free oxygen (O2), is oxygen that is not bonded to any other element. Dissolved oxygen is the presence of these free O2 molecules within water.The bonded oxygen molecule in water (H2O) is in a compound and does not count toward dissolved oxygen levels. One can imagine that free oxygen molecules dissolve in water much the way salt or sugar does when it is stirred ².
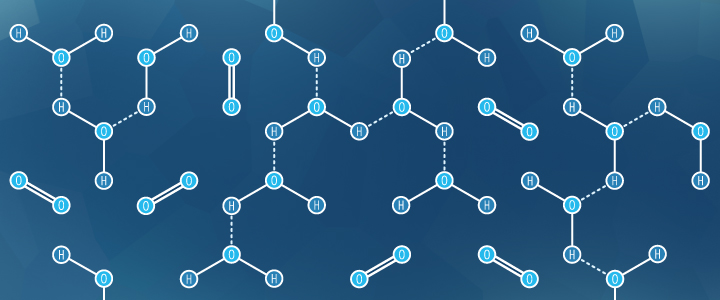
Dissolved Oxygen and Aquatic Life
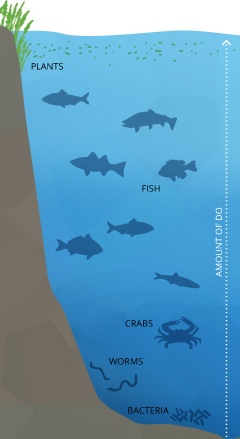
Dissolved oxygen is necessary to many forms of life including fish, invertebrates, bacteria and plants. These organisms use oxygen in respiration, similar to organisms on land. Fish and crustaceans obtain oxygen for respiration through their gills, while plant life and phytoplankton require dissolved oxygen for respiration when there is no light for photosynthesis 4. The amount of dissolved oxygen needed varies from creature to creature. Bottom feeders, crabs, oysters and worms need minimal amounts of oxygen (1-6 mg/L), while shallow water fish need higher levels (4-15 mg/L)⁵.
Microbes such as bacteria and fungi also require dissolved oxygen. These organisms use DO to decompose organic material at the bottom of a body of water. Microbial decomposition is an important contributor to nutrient recycling. However, if there is an excess of decaying organic material (from dying algae and other organisms), in a body of water with infrequent or no turnover (also known as stratification), the oxygen at lower water levels will get used up quicker ⁶.
Where Does DO Come From?
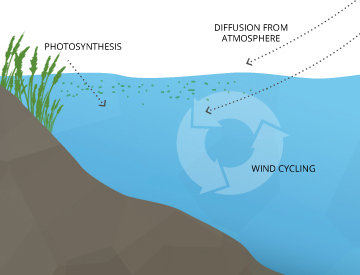
Dissolved oxygen enters water through the air or as a plant byproduct. From the air, oxygen can slowly diffuse across the water’s surface from the surrounding atmosphere, or be mixed in quickly through aeration, whether natural or man-made 7. The aeration of water can be caused by wind (creating waves), rapids, waterfalls, ground water discharge or other forms of running water. Man-made causes of aeration vary from an aquarium air pump to a hand-turned waterwheel to a large dam.
Dissolved oxygen is also produced as a waste product of photosynthesis from phytoplankton, algae, seaweed and other aquatic plants 8.
Dissolved Oxygen from Photosynthesis
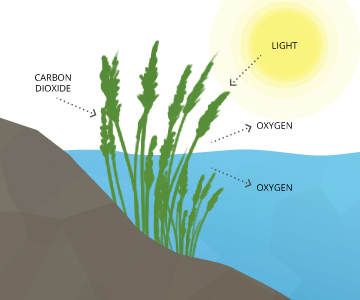
While most photosynthesis takes place at the surface (by shallow water plants and algae), a large portion of the process takes place underwater (by seaweed, sub-surface algae and phytoplankton). Light can penetrate water, though the depth that it can reach varies due to dissolved solids and other light-scattering elements present in the water. Depth also affects the wavelengths available to plants, with red being absorbed quickly and blue light being visible past 100 m. In clear water, there is no longer enough light for photosynthesis to occur beyond 200 m, and aquatic plants no longer grow. In turbid water, this photic (light-penetrating) zone is often much shallower.
Regardless of wavelengths available, the cycle doesn’t change ⁹. In addition to the needed light, CO2 is readily absorbed by water (it’s about 200 times more soluble than oxygen) and the oxygen produced as a byproduct remains dissolved in water¹⁰. The basic reaction of aquatic photosynthesis remains:
CO2 + H2O → (CH2O) + O2
As aquatic photosynthesis is light-dependent, the dissolved oxygen produced will peak during daylight hours and decline at night ⁸.
Dissolved Oxygen Saturation
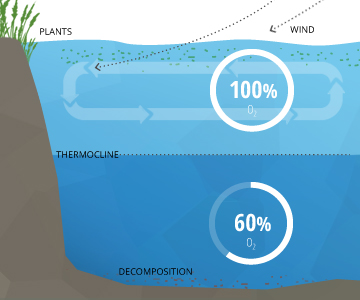
In a stable body of water with no stratification, dissolved oxygen will remain at 100% air saturation. 100% air saturation means that the water is holding as many dissolved gas molecules as it can in equilibrium. At equilibrium, the percentage of each gas in the water would be equivalent to the percentage of that gas in the atmosphere – i.e. its partial pressure ¹³. The water will slowly absorb oxygen and other gasses from the atmosphere until it reaches equilibrium at complete saturation 10. This process is sped up by wind-driven waves and other sources of aeration ³.
In deeper waters, DO can remain below 100% due to the respiration of aquatic organisms and microbial decomposition. These deeper levels of water often do not reach 100% air saturation equilibrium because they are not shallow enough to be affected by the waves and photosynthesis at the surface ³. This water is below an invisible boundary called the thermocline (the depth at which water temperature begins to decline)¹¹.
What Affects Oxygen Solubility?
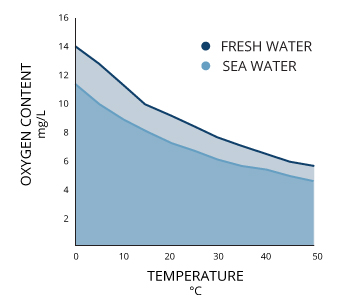
Two bodies of water that are both 100% air-saturated do not necessarily have the same concentration of dissolved oxygen. The actual amount of dissolved oxygen (in mg/L) will vary depending on temperature, pressure and salinity ¹.
First, the solubility of oxygen decreases as temperature increases ¹. This means that warmer surface water requires less dissolved oxygen to reach 100% air saturation than does deeper, cooler water. For example, at sea level (1 atm or 760 mmHg) and 4°C (39°F), 100% air-saturated water would hold 10.92 mg/L of dissolved oxygen. ³ But if the temperature were raised to room temperature, 21°C (70°F), there would only be 8.68 mg/L DO at 100% air saturation ³.
Second dissolved oxygen decreases exponentially as salt levels increase ¹. That is why, at the same pressure and temperature, saltwater holds about 20% less dissolved oxygen than freshwater ³.
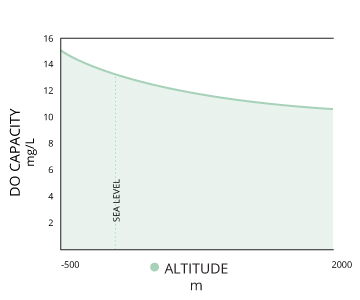
Third, dissolved oxygen will increase as pressure increases ¹. This is true of both atmospheric and hydrostatic pressures. Water at lower altitudes can hold more dissolved oxygen than water at higher altitudes. This relationship also explains the potential for “supersaturation” of waters below the thermocline – at greater hydrostatic pressures, water can hold more dissolved oxygen without it escaping ¹. Gas saturation decreases by 10% per meter increase in depth due to hydrostatic pressure ¹². This means that if the concentration of dissolved oxygen is at 100% air saturation at the surface, it would only be at 70% air saturation three meters below the surface.
In summary, colder, deeper fresh waters have the capability to hold higher concentrations of dissolved oxygen, but due to microbial decomposition, lack of atmospheric contact for diffusion and the absence of photosynthesis, actual DO levels are often far below 100% saturation ¹⁰. Warm, shallow saltwater reaches 100% air saturation at a lower concentration, but can often achieve levels over 100% due to photosynthesis and aeration. Shallow waters also remain closer to 100% saturation due to atmospheric contact and constant diffusion ¹⁰.
If there is a significant occurrence of photosynthesis or a rapid temperature change, the water can achieve DO levels over 100% air saturation. At these levels, the dissolved oxygen will dissipate into the surrounding water and air until it levels out at 100% ³.
How Can Water be More than 100% Saturated?
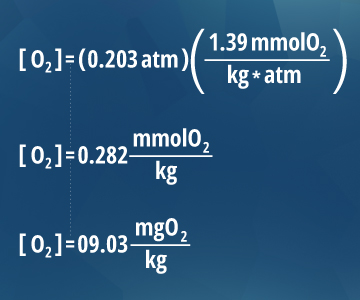
100% air saturation is the equilibrium point for gases in water. This is because gas molecules diffuse between the atmosphere and the water’s surface. According to Henry’s Law, the dissolved oxygen content of water is proportional to the percent of oxygen (partial pressure) in the air above it 13. As oxygen in the atmosphere is about 20.3%, the partial pressure of oxygen at sea level (1 atm) is 0.203 atm. Thus the amount of dissolved oxygen at 100% saturation at sea level at 20° C is 9.03 mg/L ¹⁰.
The equation shows that water will remain at 100% air saturation at equilibrium. However, there are several factors that can affect this. Aquatic respiration and decomposition lower DO concentrations, while rapid aeration and photosynthesis can contribute to supersaturation. During the process of photosynthesis, oxygen is produced as a waste product. This adds to the dissolved oxygen concentration in the water, potentially bringing it above 100% saturation ¹⁴. In addition, the equalization of water is a slow process (except in highly agitated or aerated situations). This means that dissolved oxygen levels can easily be more than 100% air saturation during the day in photosynthetically active bodies of water ¹⁴.
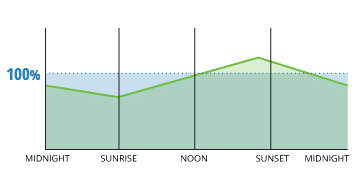
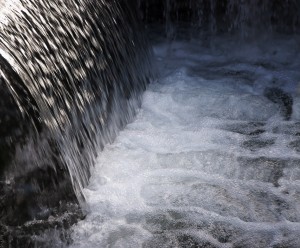
Supersaturation caused by rapid aeration is often seen beside hydro-power dams and large waterfalls ¹². Unlike small rapids and waves, the water flowing over a dam or waterfall traps and carries air with it, which is then plunged into the water. At greater depths and thus greater hydrostatic pressures, this entrained air is forced into solution, potentially raising saturation levels over 100% ¹².
Rapid temperature changes can also create DO readings greater than 100% ¹⁴. As water temperature rises, oxygen solubility decreases. On a cool summer night, a lake’s temperature might be 60° F. At 100% air saturation, this lake’s dissolved oxygen levels would be at 9.66 mg/L. When the sun rises and warms up the lake to 70° F, 100% air saturation should equate to 8.68 mg/L DO ³. But if there is no wind to move the equilibration along, the lake will still contain that initial 9.66 mg/L DO, an air saturation of 111%.
Typical Dissolved Oxygen Levels
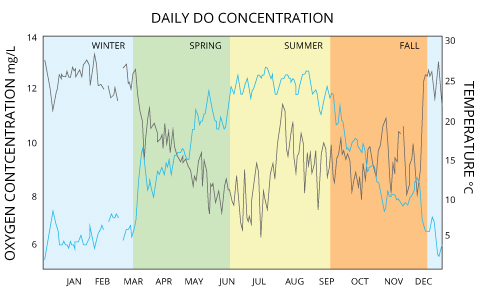
Dissolved oxygen concentrations are constantly affected by diffusion and aeration, photosynthesis, respiration and decomposition. While water equilibrates toward 100% air saturation, dissolved oxygen levels will also fluctuate with temperature, salinity and pressure changes ³. As such, dissolved oxygen levels can range from less than 1 mg/L to more than 20 mg/L depending on how all of these factors interact. In freshwater systems such as lakes, rivers and streams, dissolved oxygen concentrations will vary by season, location and water depth.
Freshwater Fluctuations: Example 1
In the Pompton River in New Jersey, mean dissolved oxygen concentrations range from 12-13 mg/L in winter and drop to 6-9 mg/L in the summer ⁸. That same river shows daily fluctuations of up to 3 mg/L due to photosynthesis production ⁸.
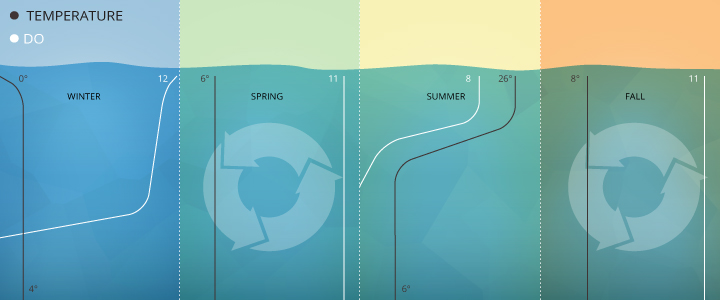
Freshwater Fluctuations: Example 2
Studies at Crooked Lake in Indiana show dissolved oxygen concentrations vary by season and depth from 12 mg/L (surface, winter) to 0 mg/L (32 m depth, late summer), with full lake turnovers in spring and fall equalizing DO levels around 11 mg/L for all depths ¹.
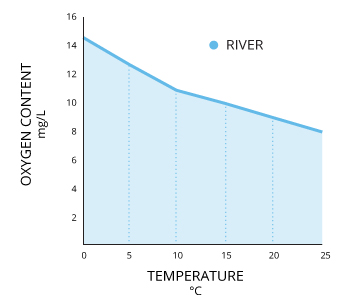
Rivers and streams tend to stay near or slightly above 100% air saturation due to relatively large surface areas, aeration from rapids, and groundwater discharge, which means that their dissolved oxygen concentrations will depend on the water temperature ¹. While groundwater usually has low DO levels, groundwater-fed streams can hold more oxygen due to the influx of colder water and the mixing it causes ¹⁵. Standard Methods for the Examination of Water and Wastewater defines dissolved oxygen in streams as the sum of photosynthetic byproducts, respiration, re-aeration, accrual from groundwater inflow and surface runoff ¹³.
Saltwater holds less oxygen than freshwater, so oceanic DO concentrations tend to be lower than those of freshwater. In the ocean, surface water mean annual DO concentrations range from 9 mg/L near the poles down to 4 mg/L near the equator with lower DO levels at further depths. There are lower dissolved oxygen concentrations near the equator because salinity is higher ¹⁶.

Some states have Water Quality Standard Acts, requiring minimum concentrations of dissolved oxygen; in Michigan, these minimums are 7 mg/L for cold-water fisheries and 5 mg/L for warm-water fish 17; in Colorado, “Class 1 Cold Water Aquatic Life” needs 6 mg/L, and “Class 1 Warm Water Aquatic Life” requires DO levels of at least 5 mg/L 15. In order to mimic ideal environmental systems, freshwater tanks ideally need around 8 mg/L DO for optimum growth and marine tank requirements range from 6-7 mg/L DO based on the salinity level ¹⁸. In other words, dissolved oxygen should be near 100% air saturation.
Examples of Freshwater Organisms and Dissolved Oxygen Requirements
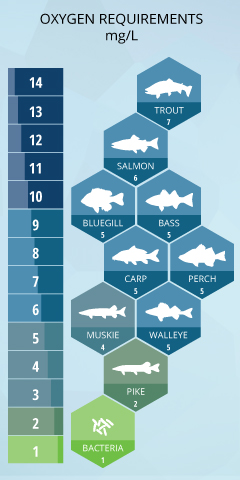
Coldwater fish like trout and salmon are most affected by low dissolved oxygen levels 19. The mean DO level for adult salmonids is 6.5 mg/L, and the minimum is 4 mg/L ¹². These fish generally attempt to avoid areas where dissolved oxygen is less than 5 mg/L and will begin to die if exposed to DO levels less than 3 mg/L for more than a couple days ¹⁹. For salmon and trout eggs, dissolved oxygen levels below 11 mg/L will delay their hatching, and below 8 mg/L will impair their growth and lower their survival rates. ¹⁹ When dissolved oxygen falls below 6 mg/L (considered normal for most other fish), the vast majority of trout and salmon eggs will die. ¹⁹
Bluegill, Largemouth Bass, White Perch, and Yellow Perch are considered warmwater fish and depend on dissolved oxygen levels above 5 mg/L21. They will avoid areas where DO levels are below 3 mg/L, but generally do not begin to suffer fatalities due to oxygen depletion until levels fall below 2 mg/L 22. The mean DO levels should remain near 5.5 mg/L for optimum growth and survival ¹².
Walleye also prefer levels over 5 mg/L, though they can survive at 2 mg/L DO levels for a short time.²⁴ Muskie need levels over 3 mg/L for both adults and eggs ²⁵. Carp are hardier, and while they can enjoy dissolved oxygen levels above 5 mg/L, they easily tolerate levels below 2 mg/L and can survive at levels below 1 mg/L ²⁶.
The freshwater fish most tolerant to DO levels include fathead minnows and northern pike. Northern pike can survive at dissolved oxygen concentrations as low as 0.1 mg/L for several days, and at 1.5 mg/L for an infinite amount of time ²⁷. Fathead minnows can survive at 1 mg/L for an extended period with only minimal effects on reproduction and growth.
As for bottom-dwelling microbes, DO changes don’t bother them much. If all the oxygen at their water level gets used up, bacteria will start using nitrate to decompose organic matter, a process known as denitrification. If all of the nitrogen is spent, they will begin reducing sulfate ¹⁷. If organic matter accumulates faster than it decomposes, sediment at the bottom of a lake simply becomes enriched by the organic material. ²⁸.
Examples of Saltwater Organisms and Dissolved Oxygen Requirements
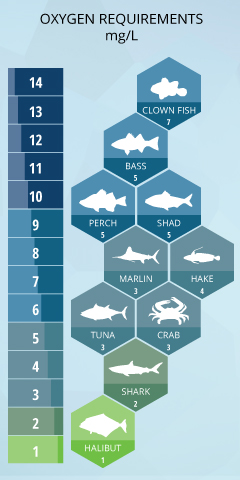
Saltwater fish and organisms have a higher tolerance for low dissolved oxygen concentrations as saltwater has a lower 100% air saturation than freshwater. In general, dissolved oxygen levels are about 20% less in seawater than in freshwater ³.
This does not mean that saltwater fish can live without dissolved oxygen completely. Striped bass, white perch and American shad need DO levels over 5 mg/L to grow and thrive ⁵. The red hake is also extremely sensitive to dissolved oxygen levels, abandoning its preferred habitat near the seafloor if concentrations fall below 4.2 mg/L ²⁹.
The dissolved oxygen requirements of open-ocean and deep-ocean fish are a bit harder to track, but there have been some studies in the area. Billfish swim in areas with a minimum of 3.5 mg/L DO, while marlins and sailfish will dive to depths with DO concentrations of 1.5 mg/L ³⁰. Likewise, white sharks are also limited in dive depths due to dissolved oxygen levels (above 1.5 mg/L), though many other sharks have been found in areas of low DO ³³. Tracked swordfish show a preference for shallow water during the day, basking in oxygenated water (7.7 mg/L) after diving to depths with concentrations around 2.5 mg/L ³⁴. Albacore tuna live in mid-ocean levels, and require a minimum of 2.5 mg/L ³⁵, while halibut can maintain a minimum DO tolerance threshold of 1 mg/L ³⁶.
Many tropical saltwater fish, including clown fish, angel fish and groupers require higher levels of DO, such as those surrounding coral reefs. Coral reefs are found in the euphotic zone (where light penetrates the water – usually not deeper than 70 m). Higher dissolved oxygen concentrations are generally found around coral reefs due to photosynthesis and aeration from eddies and breaking waves ³⁷. These DO levels can fluctuate from 4-15 mg/L, though they usually remain around 5-8 mg/L, cycling between day photosynthesis production and night plant respiration ³⁸. In terms of air saturation, this means that dissolved oxygen near coral reefs can easily range from 40-200% ³⁹.
Crustaceans such as crabs and lobsters are benthic (bottom-dwelling) organisms, but still require minimum levels of dissolved oxygen. Depending on the species, minimum DO requirements can range from 4 mg/L to 1 mg/L ¹³. Despite being bottom dwellers, mussels, oysters and clams also require a minimum of 1-2 mg/L of dissolved oxygen 29, which is why they are found in shallower, coastal waters that receive oxygen from the atmosphere and photosynthetic sources.
Consequences of Unusual DO Levels
If dissolved oxygen concentrations drop below a certain level, fish mortality rates will rise. Sensitive freshwater fish like salmon can’t even reproduce at levels below 6 mg/L ¹⁹. In the ocean, coastal fish begin to avoid areas where DO is below 3.7 mg/L, with specific species abandoning an area completely when levels fall below 3.5 mg/L ²⁹. Below 2.0 mg/L, invertebrates also leave and below 1 mg/L even benthic organisms show reduced growth and survival rates ²⁹.
Fish kill / Winterkill
A fishkill occurs when a large number of fish in an area of water die off. It can be species-based or a water-wide mortality. Fish kills can occur for a number of reasons, but low dissolved oxygen is often a factor. A winterkill is a fish kill caused by prolonged reduction in dissolved oxygen due to ice or snow cover on a lake or pond ²⁰.
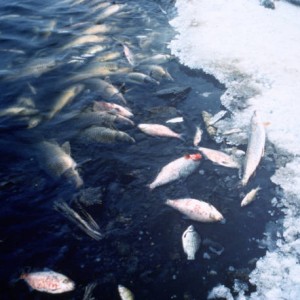
When a body of water is overproductive, the oxygen in the water may get used up faster than it can be replenished. This occurs when a body of water is overstocked with organisms or if there is a large algal bloom die-off.
Fish kills are more common in eutrophic lakes: lakes with high concentrations of nutrients (particularly phosphorus and nitrogen) ⁴¹. High levels of nutrients fuel algae blooms, which can initially boost dissolved oxygen levels. But more algae means more plant respiration, drawing on DO, and when the algae die, bacterial decomposition spikes, using up most or all of the dissolved oxygen available. This creates an anoxic, or oxygen-depleted, environment where fish and other organisms cannot survive. Such nutrient levels can occur naturally, but are more often caused by pollution from fertilizer runoff or poorly treated wastewater ⁴¹.
Winterkills occur when respiration from fish, plants and other organisms is greater than the oxygen production by photosynthesis ¹. They occur when the water is covered by ice, and so cannot receive oxygen by diffusion from the atmosphere. If the ice is then covered by snow, photosynthesis also cannot occur, and the algae will depend entirely on respiration or die off. In these situations, fish, plants and decomposition are all using up the dissolved oxygen, and it cannot be replenished, resulting in a winter fish kill. The shallower the water, and the more productive (high levels of organisms) the water, the greater the likelihood of a winterkill ²⁰.
Gas Bubble Disease
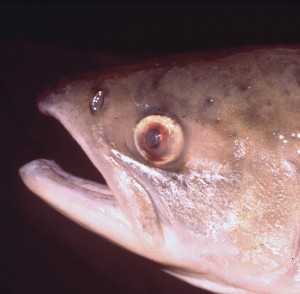
Just as low dissolved oxygen can cause problems, so too can high concentrations. Supersaturated water can cause gas bubble disease in fish and invertebrates ¹². Significant death rates occur when dissolved oxygen remains above 115%-120% air saturation for a period of time. Total mortality occurs in young salmon and trout in under three days at 120% dissolved oxygen saturation ¹². Invertebrates, while also affected by gas bubble disease, can usually tolerate higher levels of supersaturation than fish ¹².
Extended periods of supersaturation can occur in highly aerated waters, often near hydropower dams and waterfalls, or due to excessive photosynthetic activity. Algae blooms can cause air saturations of over 100% due to large amounts of oxygen as a photosynthetic byproduct. This is often coupled with higher water temperatures, which also affects saturation. ¹² At higher temperatures, water becomes 100% saturated at lower concentrations, so higher dissolved oxygen concentrations mean even higher air saturation levels.
Dead Zones
A dead zone is an area of water with little to no dissolved oxygen present. They are so named because aquatic organisms cannot survive there. Dead zones often occur near heavy human populations, such as estuaries and coastal areas off the Gulf of Mexico, the North Sea, the Baltic Sea, and the East China Sea. They can occur in large lakes and rivers as well, but are more well known in the oceanic context.
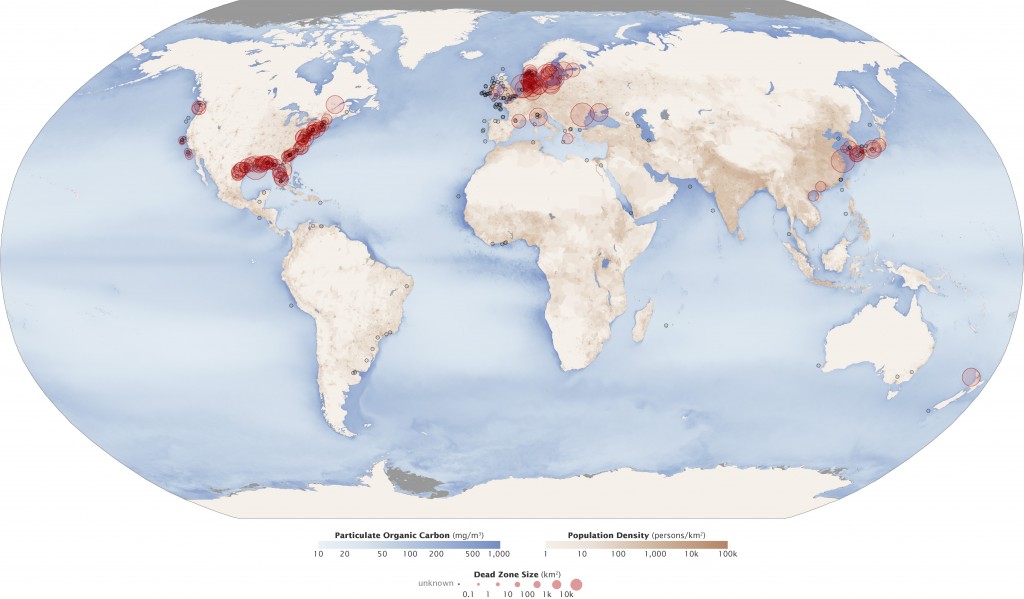
These zones are usually a result of a fertilizer-fueled algae and phytoplankton growth boom. When the algae and phytoplankton die, the microbes at the seafloor use up the oxygen decomposing the organic matter ³¹. These anoxic conditions are usually stratified, occurring only in the lower layers of the water. While some fish and other organisms can escape, shellfish, young fish and eggs usually die ³².
Naturally occurring hypoxic (low oxygen) conditions are not considered dead zones. The local aquatic life (including benthic organisms) have adjusted to the recurring low-oxygen conditions, so the adverse effects of a dead zone (mass fish kills, sudden disappearance of aquatic organisms, and growth/development problems in fish and invertebrates) do not occur ³¹.
Such naturally occurring zones frequently occur in deep lake basins and lower ocean levels due to water column stratification.
Dissolved Oxygen and Water Column Stratification
Stratification separates a body of water into layers. This layering can be based on temperature or dissolved substances (namely salt and oxygen) with both factors often playing a role. The stratification of water has been commonly studied in lakes, though it also occurs in the ocean. It can also occur in rivers if pools are deep enough and in estuaries where there is a significant division between freshwater and saltwater sources.
Lake Stratification
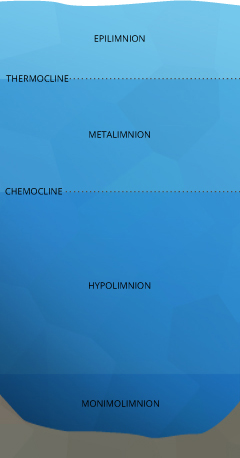
The uppermost layer of a lake, known as the epilimnion, is exposed to solar radiation and contact with the atmosphere, keeping it warmer. The depth of the epilimnion is dependent on the temperature exchange, usually determined by water clarity and depth of mixing (usually initiated by wind) ¹¹. Within this upper layer, algae and phytoplankton engage in photosynthesis. Between the contact with the air, potential for aeration and the byproducts of photosynthesis, dissolved oxygen in the epilimnion remains near 100% saturation. The exact levels of DO vary depending on the temperature of the water, the amount of photosynthesis occurring and the quantity of dissolved oxygen used for respiration by aquatic life.
Below the epilimnion is the metalimnion, a transitional layer that fluctuates in thickness and temperature. The boundary between the epilimnion and metalimnion is called the thermocline – the point at which water temperature begins to steadily drop off ¹¹. Here, two different outcomes can occur. If light can penetrate beyond the thermocline and photosynthesis occurs in this strata, the metalimnion can achieve an oxygen maximum ¹¹. This means that the dissolved oxygen level will be higher in the metalimnion than in the epilimnion. But in eutrophic or nutrient-rich lakes, the respiration of organisms can deplete dissolved oxygen levels, creating a metalimnetic oxygen minimum ⁴².
The next layer is the hypolimnion. If the hypolimnion is deep enough to never mix with the upper layers, it is known as the monimolimnion. The hypolimnion is separated from the upper layers by the chemocline or halocline. These clines mark the boundary between oxic and anoxic water and salinity gradients, respectively. ¹¹. While lab conditions would conclude that at colder temperatures and higher pressures water can hold more dissolved oxygen, this is not always the result. In the hypolimnion, bacteria and fungi use dissolved oxygen to decompose organic material ⁶. This organic material comes from dead algae and other organisms that sink to the bottom. The dissolved oxygen used in decomposition is not replaced – there is no atmospheric contact, aeration or photosynthesis to restore DO levels in the hypolimnion ¹¹. Thus the process of decomposition “uses up” all of the oxygen within this layer.
If the lake in question is a holomictic “mixing” lake, all the layers mix at least once per year (usually spring and fall) when lake strata temperatures align. This turnover redistributes dissolved oxygen throughout all the layers and the process begins again.
Ocean Stratification
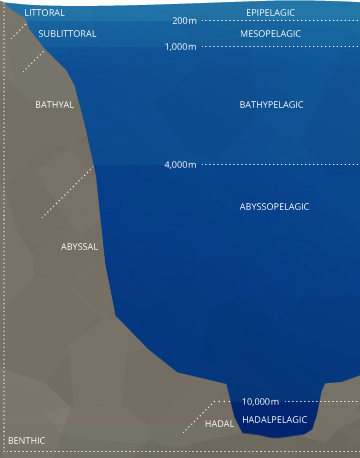
Stratification in the ocean is both horizontal and vertical. The littoral, or coastal area is most affected by estuaries and other inflow sources.⁴⁴ It tends to be shallow and tidal with fluctuating dissolved oxygen levels. The sublittoral, also known as the neritic or demersal zone, is considered a coastal zone as well. In this zone, dissolved oxygen concentrations may vary but they do not fluctuate as much as they do in the littoral zone.
This is the zone where many coral reefs grow, and DO levels remain near 100% air saturation due to eddies, breaking waves and photosynthesis 45. This zone is also where most oceanic benthic (bottom-dwelling) organisms exist. Oceanic benthic fish do not live at the greatest depths of the ocean. They dwell at the seafloor near to coasts and oceanic shelves while remaining in the upper levels of the ocean.
Beyond the demersal zone are the bathyal, abyssal and hadal plains, which are fairly similar in terms of consistently low DO.
In the open ocean, there are five major vertical strata: epipelagic, mesopelagic, bathypelagic, abyssopelagic, and hadalpelagic ⁴⁴. The exact definitions and depths are subjective, but the following information is generally agreed upon. The epipelagic is also known as the surface layer or photic zone (where light penetrates). This is the layer with the highest levels of dissolved oxygen due to wave action and photosynthesis. The epipelagic generally reaches to 200 m and is bordered by a collection of clines.
These clines can overlap or exist at separate depths. Much like in a lake, the thermocline divides oceanic strata by temperature. The halocline divides by salinity levels and the pycnocline divides strata by density ¹⁶. Each of these clines can affect the amount of dissolved oxygen the ocean strata can hold.
The mesopelagic, meaning “twilight” zone, stretches from 200-1000 m. Depending on water clarity, some light may filter through, but there is not enough for photosynthesis to occur ⁴⁴. Within this strata, the oxygen minimum zone (OMZ) can occur. The OMZ develops because organisms use the oxygen for respiration, but it is too deep to be replenished by photosynthetic oxygen byproducts or aeration from waves. This zone tends to exist around a depth of 500 m ⁴⁵. The mesopelagic zone is bordered by chemoclines (clines based on chemistry levels, e.g. oxygen and salinity) on both sides, reflecting different dissolved oxygen and salinity levels between the strata.
Below the mesopelagic is the aphotic zone(s). These strata have lower dissolved oxygen levels than the surface water because photosynthesis does not occur but can have higher levels than the OMZ because less respiration occurs.
The bathypelagic, “midnight” zone exists between 1000-4000 m, and many creatures can still live here. The bottom layer of the ocean is the abyssopelagic, which exists below 4000 m. The hadopelagic is the name for the zone of deep ocean trenches that open below the abyssal plain, such as the Mariana Trench ⁴⁴.
Estuary Stratification
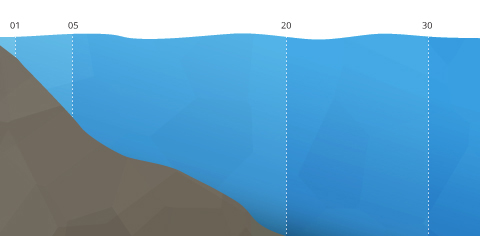
Estuary stratifications are based on salinity distributions. Because saltwater holds less dissolved oxygen than freshwater, this can affect aquatic organism distribution. The stronger the river flow, the higher the oxygen concentrations. This stratification can be horizontal, with DO levels dropping from inland to open ocean, or vertical, with the fresh, oxygenated river water floating over the low DO seawater ⁴⁶. When the stratification is clearly defined, a pycnocline divides the fresher water from the salt water, contributing to separate dissolved oxygen concentrations in each strata.
Dissolved Oxygen Units and Reporting
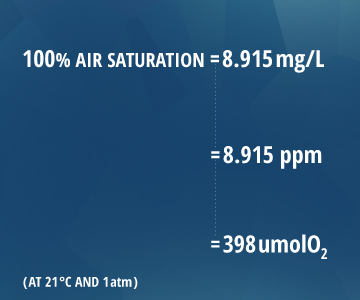
Dissolved oxygen is usually reported in milligrams per liter (mg/L) or as a percent of air saturation. However, some studies will report DO in parts per million (ppm) or in micromoles (umol). 1 mg/L is equal to 1 ppm. The relationship between mg/L and % air saturation has been discussed above, and varies with temperature, pressure and salinity of the water. One micromole of oxygen is equal to 0.022391 milligrams, and this unit is commonly used in oceanic studies ⁴⁷. Thus 100 umol/L O2 is equal to 2.2 mg/L O2.
Calculating DO from % Air Saturation
To calculate dissolved oxygen concentrations from air saturation, it is necessary to know the temperature and salinity of the sample. Barometric pressure has already been accounted for as the partial pressure of oxygen contributes to the percent air saturation 7. Salinity and temperature can then be used in Henry’s Law to calculate what the DO concentration would be at 100% air saturation 10. However, it is easier to use an oxygen solubility chart. These charts show the dissolved oxygen concentration at 100% air saturation at varying temperatures, and salinities. This value can then be multiplied by the measured percent air saturation to calculate the dissolved oxygen concentration 7.
O2 mg/L = (Measured % DO)*(DO value from chart at temperature and salinity)
Example:
70% DO measured
35 ppt salinity
15°C
.70 * 8.135 = 5.69 mg/L DO
Cite This Work
Fondriest Environmental, Inc. “Dissolved Oxygen.” Fundamentals of Environmental Measurements. 19 Nov. 2013. Web. < https://www.fondriest.com/environmental-measurements/parameters/water-quality/dissolved-oxygen/ >.